The concept of aperiodic geometry has existed for at least 1000 years, from Islamic mosaics and mosaics to ancient inlay and silkwork patterns. Since ancient times, artists and geometers have thought about how to place shapes on a plane that never overlaps or repeats. To illustrate this not-so-periodic moiré geometry (French for “waves”), two identical periodic patterns are superimposed, but one pattern is slightly shifted, rotated, or stretched..
Like optical illusions, non-periodic moiré patterns create a smooth imitation of motion; As engineering tools, they are often used in fields of view such as optics, photography and color printing, as well as in seemingly distant fields such as marine biology or the detection of counterfeit banknotes. And at the atomic scale, moire patterns can produce some unusual electrical properties.
An example of the muare effect (University of Utah)
Mathematicians from the University of Utah discoveredthat by bending one square lattice over another, composite materials based on a two-layer moire pattern exhibit electrical and physical properties that can vary quite dramatically. His inventions resonate twisttronicsthe science of bending atomic lattices and some rather complex geometric principles.
The discovery could have implications for many industries, as engineers can precisely calibrate the electrical, optical, thermal, and even acoustic properties of these materials. In particular, twisttronic and non-periodic geometry could soon illuminate the path to high-temperature superconductivity, revolutionize electronic devices and perhaps even accelerate the emergence of quantum computers.
“We rotated and widened two regular cages relative to each other, creating a veritable zoo of microgeometry, and amazing patterns emerged,” says Ken Golden, distinguished professor of mathematics at the University of Utah and senior author of the study. “The resulting moire is a template for the geometric arrangement of the two constituent materials, which together form a new spun bilayer composite,” he says. Popular Mechanics. Consider piles of wire stacked on top of each other; they can bend relative to each other and form entirely new moiré scales of periodicity or non-periocity.
Periodic geometries have distinct patterns that repeat at regular intervals – for example, an infinite grid of squares on a checkerboard, hexagons in honeycombs, or the molecular structure of table salt. In contrast, quasi-periodic behavior is a repetition pattern that may reflect a marked regularity or a component of unpredictability or randomness. Climate fluctuations such as El Niño are quasi-periodic because they can occur regularly under the influence of external forcing (called “frequency locked modes”), but the time intervals between these events are rough estimates. These functions can mimic regularity or randomness, which are ingenious shape-shifting mathematical chameleons.
While periodic geometry is ordered and determined by a very specific recipe, and quasi-periodic models sometimes mimic regularity, non-periodic geometry is a true transition to chaos. The Penrose program (named after Roger Penrose, the mathematician and physicist who studied them in the 1970s) is a classic example. A Penrose mosaic is a plane covered with non-overlapping polygons; these patterns are not periodic because if a Penrose tile is moved any finite distance without turning, the same tile can never be reproduced.
Typically, such mosaics are predictable, but they lack translational symmetry (meaning that the shifted copy will never match the original). Glass and certain types of crystals also contain nonperiodic atomic structures: examples of natural nonperiodic geometry. Semi-crystalline alloys, in particular, Welding
Source: Port Altele
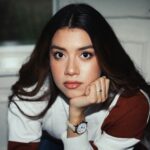