UCLA Health researchers discovered a process that remembers sleep while reducing metabolic costs even during sleep. This effective memory is located in the part of the brain that is important for learning and memory and where Alzheimer’s disease occurs. The discovery was published in a journal Nature Communication.
Sounds familiar: You go to the kitchen to get something, but when you get there you forget what you wanted. This is a failure of your working memory. Working memory is defined as remembering some information for a short period of time while busy with other activities. We use working memory almost all the time. Patients with Alzheimer’s disease and dementia have working memory impairments, which are also manifested in mild cognitive impairment (MCI). Therefore, significant efforts have been devoted to understanding the mechanisms by which large networks of neurons in the brain create working memory.
Role of the entorhinal cortex
During working memory tasks, the outer layer of the brain, known as the neocortex, sends sensory information to deeper parts of the brain, including a central area called the entorhinal cortex, which is critical for forming memories. Neurons in the entorhinal cortex exhibit a complex set of responses that have long puzzled scientists and led to the 2014 Nobel Prize in Medicine, but the mechanisms driving this complexity are unknown. The entorhinal cortex is where Alzheimer’s disease begins to occur.
“Therefore, it is crucial to understand what kind of magic happens in the cortico-entorhinal network when the neocortex communicates with the entorhinal cortex, which converts it into working memory. This could enable early diagnosis of Alzheimer’s disease and related dementias, as well as mild cognitive impairments,” said the neurophysicist and corresponding author Mayank Mehta, director of the WM Keck Center for Neurophysics and the Center for the Physics of Life at UCLA.
To solve this problem, Mehta and his co-authors invented a new approach: the “mathematical microscope.”
In the world of physics, from Kepler to Newton to Einstein, mathematical models are routinely used to reveal surprising things we have never seen before or even imagined, such as the inner workings of subatomic particles and the inside of a black hole. Mathematical models are also used in brain sciences, but their predictions are not taken as seriously as in physics. This is because in physics, the predictions of mathematical theories are tested not only qualitatively but also quantitatively.
It is generally believed that such quantitatively precise experimental tests of mathematical theories are impossible in biology because the brain is much more complex than the physical world. Mathematical theories in physics are very simple, involve very few free parameters, and therefore require precise experimental tests. In contrast, the brain has billions of neurons and trillions of connections; It is a mathematical nightmare, let alone high-precision microscopy.
Simplifying complex systems
“To solve this seemingly impossible task of developing a simple theory that can explain experimental data on in vivo memory dynamics with high fidelity, we hypothesized that cortico-entorhinal dialogue and memory magic would occur even when the sub-subjects were asleep or under anesthesia,” said the study’s lead author, Dr. Krishna Choudhary. “Just like a car behaves like a car when it’s idling or going 70 miles per hour.”
Then researchers from the University of California, Los Angeles made another important assumption: The dynamics of the entire cortex and entorhinal cortex during sleep or anesthesia can be captured by just two neurons. These assumptions reduced the problem of billions of neuronal interactions to just two free variables: the strength of the input from the neocortex to the entorhinal cortex and the strength of recurrent connections within the entorhinal cortex. Although this makes the problem mathematically solvable, the obvious question is: is this true?
“If we test our theory quantitatively with in vivo data, we find that this is just fun math games, not a clear understanding of the magic of memory creation,” Mehta said.
Decisive experimental tests of this theory were carried out by co-author Dr. It required complex experiments by Thomas Hahn.
“The entorhinal cortex is a complex circuit. To truly test the theory, we needed experimental methods that not only measure neuronal activity with high precision but also determine the exact anatomical location of the neuron,” Hahn said.
Hahn, also one of the co-authors, and Dr. Sven Berberich measured the membrane potential of identified entorhinal cortex neurons in vivo using the whole-cell clamp technique and then used anatomical techniques to identify the neuron. They also measured the activity of the parietal cortex, the part of the neocortex that sends data to the entorhinal cortex.
“Mathematical theory and complex in vivo data are necessary and wonderful, but we needed to solve another problem: How do we match this simple theory with complex neural data?” Mehta said.
“It took a long development process to create a ‘mathematical microscope’ that could directly reveal the inner workings of neurons as they form a memory,” Choudhary said. “As far as we know, this hasn’t been done before.”
Discovery of new memory states
The authors observed that signals from the neocortex intermittently oscillate between on and off states while a person or animal sleeps, similar to an ocean wave that builds and then breaks on the shore. Meanwhile, the entorhinal cortex was moving like a swimmer in water; When the waves rose, they could move up, and when they receded, they could go down. The data showed that and the model captured that. But using this simple coincidence, Mehta said, the model took on a life of its own, spontaneously revealing a new type of memory state known as persistent immobility.
“It’s like a wave is coming and the entorhinal cortex says: ‘No wave! I’ll say there hasn’t been a wave recently, so I’ll ignore this current wave and make no response.’ “This is persistent inertia,” Mehta said. “On the other hand, persistent activity occurs when the cortical wave weakens, but the entorhinal neurons remember that the wave is very new and continue to move forward.”
While many theories of working memory posit the existence of persistent activity, the authors found, persistent inactivity was something the model predicted and had never been observed before.
“The nice thing about sustained inactivity is that it requires almost no energy, unlike sustained activity, which requires a lot of energy,” Mehta said. “Twice the cost of metabolic energy.”
Dr. “It sounded too good to be true, so we really pushed our mathematical microscope to its limits, a regime it wasn’t designed to work in,” Choudhary said. “If the microscope were accurate, it would continue to work perfectly even in unusual situations.”
“The mathematical microscope made a dozen predictions not only for the entorhinal region but also for many other areas of the brain. We were amazed to find that the mathematical microscope worked every time,” Mehta continued. “Such a perfect match between the predictions of mathematical theory and experiments is unprecedented in neuroscience.
“This mathematical model, which matches the experiments perfectly, is a new microscope,” Mehta continued. “It reveals things that no existing microscope can see without it. No matter how many neurons you image, it won’t detect any of them.”
“In fact, metabolic abnormalities are a common feature of many memory disorders,” Mehta said. “Mehta’s laboratory is currently continuing this work to understand how complex working memory is formed and what goes wrong in the entorhinal cortex in Alzheimer’s disease, dementia, and other memory disorders.”
Source: Port Altele
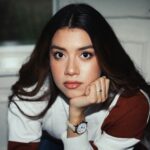